Have you ever used a dimmer on a lamp or room light and wondered how you were able to adjust the brightness of light so easily? How about a running fan in your room on a hot day? Ever wondered what it was that allowed you to change how fast the fan went? Maybe you work at an assembly plant, and see the machines moving and performing tasks with precise speed and timing. Has it ever crossed your mind as to how these giant machinations can do such complicated things and not breakdown or catastrophically collide into one another? That is the wonder known as the Variable Frequency Drive or VFD.
So WTF is a VFD?
Variable Frequency Drives (VFDs), alternatively referred to as Variable Speed Drives (VSDs) or Adjustable Frequency Drives (AFDs), constitute a fundamental aspect of industrial automation. These electronic devices are meticulously engineered to govern the speed and torque of electric motors. They achieve tha by manipulating the frequency and voltage supplied to them. By exerting control over these parameters, VFDs grant the ability to finely regulate motor speeds. This results in optimized operational efficiency and performance across a wide array of industrial applications.
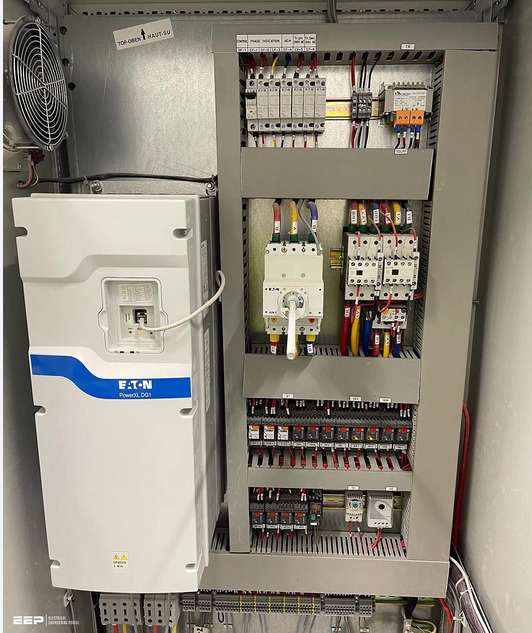
In the realm of industrial automation, VFDs offer a multifaceted range of advantages. Among their most prominent benefits is the potential for substantial energy conservation. By permitting motors to operate at lower speeds when maximum power output isn’t essential, VFDs contribute to marked reductions in power consumption, thereby fostering an environment of heightened energy efficiency. Moreover, these drives contribute significantly to the realm of process control and productivity enhancement. Through the implementation of gradual acceleration and deceleration of motors, VFDs curtail mechanical stress and wear on machinery, leading to prolonged equipment lifespan and diminished maintenance expenditures. Their capacity for intricate adjustments further facilitates seamless synchronization with other processes and components, ultimately culminating in elevated product quality and consistency within the sphere of manufacturing and production.
A Brief History in VFD’s
The history of Variable Frequency Drives (VFDs) traces back to the mid-20th century. During this time advancements in power electronics and control systems set the stage for their development. The initial concept of controlling motor speeds by varying the frequency of the power supply emerged in the 1960s. However, the state of electronics at the time limited the technology. The 1970s saw the advent of solid-state devices like thyristors and later insulated gate bipolar transistors (IGBTs). These components enabled more efficient and precise control over motor speeds by allowing the modulation of voltage and frequency.
By the 1980s, the electronics industry had made significant strides, making VFDs more practical and cost-effective. Advances in microprocessors and digital control algorithms led to the emergence of more sophisticated and versatile VFD systems. These drives gained traction in various industries where precise speed control and energy efficiency were crucial. Some examples include HVAC systems, pumps, and conveyors. The turn of the millennium saw further improvements in power electronics, enabling higher switching frequencies and greater efficiency. Additionally, advancements in communication protocols and networking facilitated seamless integration of VFDs into larger industrial automation systems.
How Are VFD’s Used
Variable Frequency Drives (VFDs) operate based on the principles of pulse width modulation (PWM) and voltage-frequency control. These drives consist of three main components: a rectifier, a DC bus, and an inverter. The process begins with the rectifier, which converts the incoming AC power supply into DC voltage. This DC voltage is then stored in the DC bus, creating a stable power source. The inverter stage is where the magic happens. The VFD generates variable frequency AC output by rapidly switching the DC voltage from the bus into a series of pulses through the inverter’s transistors. The width of these pulses determines the effective voltage and frequency delivered to the motor, effectively controlling its speed and torque.
To elaborate on the concept of pulse width modulation (PWM), imagine turning a light switch on and off very rapidly. When the switch is on, the light receives full power, and when it’s off, the light receives no power. By adjusting the duration of the on and off states, you can regulate the average power delivered to the light, thus controlling its brightness. Similarly, VFDs use PWM to regulate motor speed. The width of the output pulses corresponds to the voltage and frequency applied to the motor. By adjusting this pulse width, VFDs can provide a wide range of speeds, allowing precise control over the motor’s performance.
Voltage-frequency control is another fundamental aspect of VFD operation. The relationship between voltage and frequency needs to be maintained within certain limits to prevent overloading the motor. As the frequency changes, the VFD adjusts the voltage to maintain an appropriate ratio. For example, as the frequency is reduced to slow down the motor, the VFD decreases the voltage proportionally to ensure the motor’s torque remains consistent. Conversely, at higher frequencies, the VFD increases the voltage to sustain the motor’s torque output. This coordinated adjustment of voltage and frequency ensures the motor operates within its safe and efficient operational range, enabling seamless control over its speed and performance in various industrial applications.
What Uses a VFD?
Implementing VFDs in industrial settings involves careful planning and integration to harness their benefits effectively. First, a thorough assessment of the application’s requirements and the specific motor system is necessary. This includes considering factors like load characteristics, torque demands, and desired speed ranges. Once the appropriate VFD is selected, the installation process involves connecting the drive to the motor and the power source. It’s essential to ensure proper grounding, wiring, and following manufacturer guidelines for correct setup.
After physical installation, configuring the VFD settings becomes crucial. This involves programming parameters such as motor nameplate data, acceleration and deceleration rates, maximum and minimum speed limits, and control modes (e.g., open-loop or closed-loop control). Advanced VFDs might also offer features like communication interfaces (such as Modbus or Ethernet) to integrate with larger automation systems for centralized control and monitoring. Careful tuning of these parameters ensures that the VFD operates optimally, delivering the desired motor performance while minimizing energy consumption and wear on equipment.
Implementing VFDs also requires considerations for safety and maintenance. Properly trained personnel should handle VFD installation and configuration to prevent accidents. Regular maintenance and periodic inspections are necessary to ensure the drives and associated components are functioning correctly. Monitoring for anomalies, such as overheating or unusual noises, can help detect potential issues early and prevent costly downtime. As technology evolves, the implementation of VFDs might also involve exploring predictive maintenance strategies that leverage data analytics and sensors to anticipate maintenance needs and enhance system reliability.
Conclusion
VFDs stand as a testament to synergy between technological innovation and industrial efficiency. VFDs have transformed the way we control and optimize electric motor-driven systems. Not only do VFDs empower industries to achieve unparalleled precision and performance through dyanmic controls. They also contribute significantly to energy conservation, equipment longevity, and process refinement. As industries continue to evolve and prioritize sustainability, VFDs will undoubtedly remain at the forefront of this evolution. VFDs continue to drive innovation, enhance productivity, and leading to a more efficient and environmentally conscious industrial landscape.
Updated on August 29, 2023 by Ken Cheng